Last week we looked at how we start with the basics by studying the biology of cancer to understand it. This helps us identify cancer-associated molecules that can be targeted with new therapies.
The next step in translating research to the clinic, bench to bedside, is to test whether our findings in the lab will have the potential to become actual treatments for children with cancer. Using sophisticated robotic technology and pre-clinical model systems, our labs provide the infrastructure needed to help us find new treatments as quickly as possible.
Testing potential new treatments in the lab
Before potential new treatments go to clinical trial, preclinical research in the lab establishes the most promising candidates. Future treatments can be tested in vitro and in vivo.
In vitro testing involves growing cancer cells in culture flasks and treating them with specific cancer drugs at different doses, sometimes on their own as single agents or else in combination with other drugs. Scientists then measure how many cancer cells die and how quickly.
To automate the process and speed it up (high throughput screening), our ACRF Drug Discovery Centre can test cancer cells against tens of thousands of chemicals (called ‘compound libraries’), looking for the proverbial needle in a haystack. Sophisticated robotics allows screening of 50,000 chemicals on a particular cancer in a week. That would take a researcher a whole year. Any drugs that kill the cells are called ‘hits’ and subjected to further research.
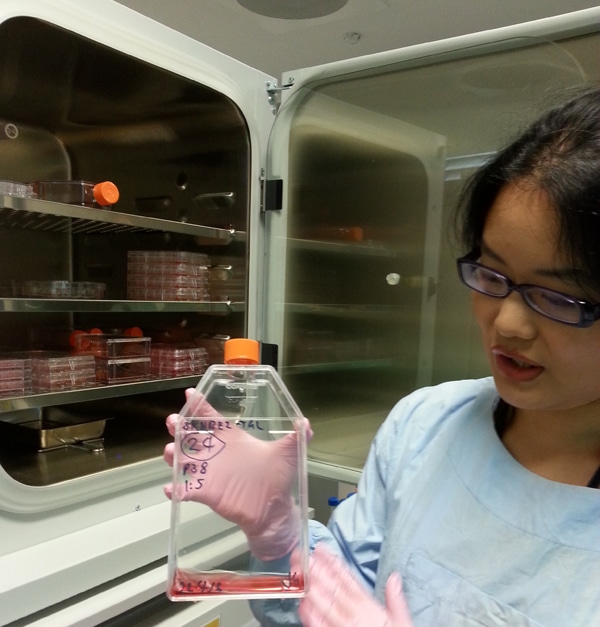
A recent drug screen found a vitamin A derivative, fenretinide, is among the few safest, most effective of 3500 drugs tested against our cultured DIPG neurospheres, the largest drug screen ever performed for the childhood brain cancer Diffuse Intrinsic Pontine Glioma (DIPG). We will gather data needed to progress fenretinide into clinical trials in the search for effective treatments for childhood brain cancer.
In vivo testing allows drugs that look promising in a drug screen to be tested in a biological model, mouse ‘avatars’, to check their safety. This is important because it ensures that only the safest, most effective drugs are tested in children.
There are a large number of drugs, developed for adult cancers, which may have promise against child cancers. However the clinical evaluation of these is hampered by small patient populations and considerable ethical considerations. Since 2005, our Leukaemia Biology laboratory headed by Professor Richard Lock has been part of the Pediatric Preclinical Testing Consortium (PPTC). The PPTC is a US National Cancer Institute-funded initiative aimed at prioritising effective drugs for clinical evaluation in paediatric cancers.
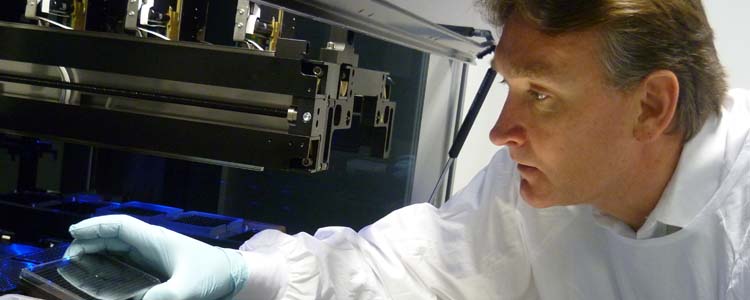
As the leukaemia testing site for the PPTC, and the only site outside the US, we use our experimental model of Acute Lymphobastic Leukaemia (ALL) to evaluate preclinical efficacy of up to 10 new drugs and drug combinations per year, with those deemed effective put forward for clinical evaluation. This has led to a number of drugs being promoted to clinical trials. Just as importantly, testing has highlighted which drugs are ineffective against paediatric ALL, helping reduce the number of ineffective agents reaching the clinic.
Effective drugs are usually investigated further in the lab, determining mechanisms of action and assessing rational combination treatments to further increase drug efficacy.
Mechanism of action
It’s one thing to know a drug kills a cancer cell but another to know how – a drug’s so-called ‘mechanism of action’. Some starve cancer cells of the nutrients they need to grow, others switch on the cancer cells’ kill-switch, yet others stop them dividing.
Our research on modified natural compound dextran-catechin revealed that it safely attacked neuroblastoma by disrupting copper metabolism. But how? Dextran-catechin disrupts a process called angiogenesis, the formation of blood vessel networks, which is dependent on copper. Dextran-catechin thus prevents tumours, which are rapidly growing, from laying down the vital supply lines that fuel cancer growth and spread – an infrastructure fail.
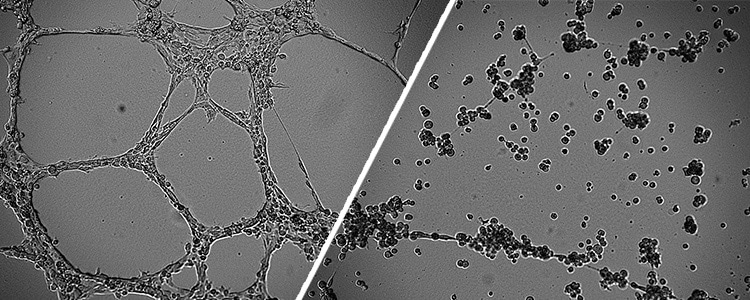
Drug repositioning
Sometimes drugs used for one disease are used to treat another – called ‘drug repositioning’ or ‘drug repurposing’.
An example of drug repositioning for childhood cancer is DFMO. This drug was used for African Sleeping Sickness originally but our researchers found it works against neuroblastoma cells grown in the lab when combined with chemotherapy. This exciting finding has since led to a clinical trial started in 2014 for children with relapsed neuroblastoma which is due to finish next year.
Next week on Awareness Wednesday, see how new treatments get into the clinic, first through clinical trials and then, if successful, as standard treatments.
Read more about the DFMO clinical trial.