Meet Thermus aquaticus
This single-celled organism, T. aquaticus for short, was first discovered in hot springs in Yellowstone National Park in the US in 1969. It’s a thermophile, meaning it’s a bacterium that loves hot temperatures. It thrives in an environment that kills nearly everything else. The discovery of T. aquaticus provided a solution to a scientific problem in the 1980s. This involved a newly emerging technique called the Polymerase Chain Reaction (PCR).
What is the Polymerase Chain Reaction (PCR)?
All cells contain DNA but in quantities too small for scientists to easily work with. PCR is a simple but ingenious process that enables scientists to increase (‘amplify’) the amounts of specific segments of DNA, by copying them over and over again. This creates enough DNA for scientists to analyse. The development of PCR earned its inventor, Kary B Mullis, the 1993 Nobel Prize in Chemistry.
How does PCR work?
DNA consists of building blocks called nucleotides. There are four different nucleotides, strung together in specific sequences to form strands, like hair. Two strands of DNA are wound around each other – the famous DNA double helix.
Step 1: Unwinding the DNA
To make copies of DNA strands, they must first be unwound. This is done by heating the DNA to 95oC which breaks the chemical bonds between the strands, unzipping the double helix.
Step 2: Signposting the DNA to be copied
The precise section of DNA to be copied is signposted using little strings of nucleotides called primers. These act as flags that indicate the starting point and the finishing line. They also ‘prime’ or initiate the copying process. Primers bind to DNA at around 55oC.
Step 3: Creating the copy
This is the assembly or extension stage. It’s done by an enzyme called a DNA polymerase, which adds (polymerises) nucleotides in the correct order, creating a copy of the template between the two primers. This process occurs at around 70oC.
Why is it called a chain reaction?
That’s the beauty of PCR. Each of the three steps above is repeated in the same order 30-50 times. With each cycle, every DNA strand that was created in the previous cycle adds to the pool of templates to be copied in the next cycle. It’s an exponential increase that, in a couple of hours, produces millions of identical copies from an initial single strand of DNA.
Where does T. aquaticus fit in?
Initially, scientists used a DNA polymerase from a bacterium they regularly worked with, a human gut bacterium called Escherichia coli or E. coli. That did the job fine, but the problem arose when the cycle was repeated. Heating the reaction to 95oC again to separate the DNA strands (template and copy) destroyed the E. coli polymerase. Fresh polymerase had to be added for every cycle. But the polymerase from heat-loving T. aquaticus is not inactivated at 95oC. It can be added just once and then work in every cycle. What was needed next was an automated machine to repeatedly cycle through the required temperatures. The result is a technique that has become standard practice in labs world-wide.
How childhood cancer researchers use PCR
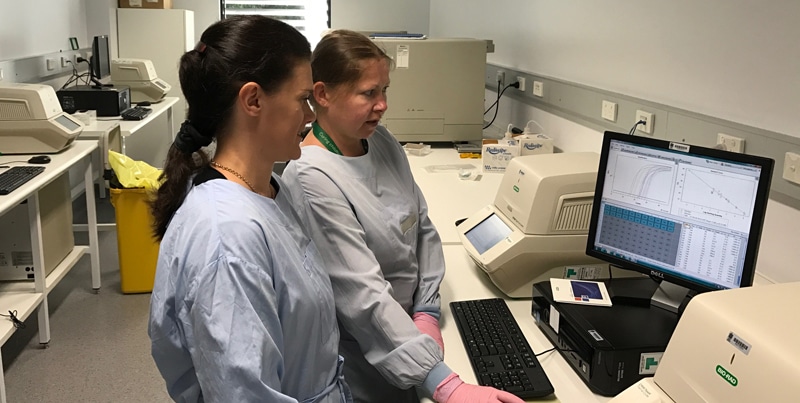
Our Minimal Residual Disease group uses PCR technology to monitor the responses of childhood leukaemia patients to treatment by looking for specific cancer cell DNA. The technique is so sensitive that it can detect one leukaemia cell lurking among 100,000 bone marrow or blood cells. Results are provided to clinicians, who modify children’s treatments if needed.
PCR is also a standard tool used by many of our scientists in their research. It’s hard to imagine a modern life sciences lab that doesn’t have multiple PCR machines like the ones in the photo above.
Steamy T. aquaticus isn’t the only little creature that’s fuelled scientific innovation. Read about how we use a firefly gene to make leukaemia cells glow to find hidden reservoirs of cancer.
Top image: Hot springs like these near Rotorua, NZ are home to thermophilic (heat-loving) bacteria like the microbe that makes PCR possible.